Hydrogen Storage Solutions for Energy Decarbonization
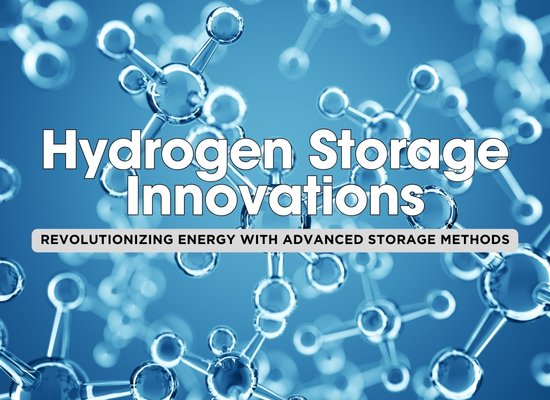
đź’ˇ Hydrogen has emerged as a game-changer in the global transition to a low-carbon energy future. As one of the most versatile energy carriers, hydrogen can power industries, fuel transportation, and store renewable energy. However, its full potential hinges on solving a critical challenge: storage. Safe, efficient, and scalable hydrogen storage solutions are essential to unlocking its role in decarbonization.
Compressed Hydrogen Storage – The Basics and Beyond
🔧 Compressed hydrogen storage is one of the most established and widely used methods of storing hydrogen, but it’s far from perfect. Let’s explore its fundamentals, current applications, and future innovations.
How It Works
- Compression Process: Hydrogen gas is compressed to pressures of 350–700 bar (5,000–10,000 psi) to reduce its volume, making it easier to store and transport.
- Storage Containers: High-pressure tanks, typically made from advanced composite materials, are used to safely contain the compressed hydrogen.
Current Applications
- Fuel Cell Vehicles: Compressed hydrogen is the primary storage method for fuel cell vehicles (FCVs) like buses, trucks, and passenger cars.
- Industrial Use: Many industrial facilities store compressed hydrogen for processes like ammonia production and metal refining.
Advantages
- Proven Technology: This method is well-understood and widely deployed.
- Scalability: Systems can be designed for a range of sizes, from small-scale vehicles to large industrial tanks.
- Fast Refueling: Compressed hydrogen allows for rapid refueling, critical for commercial transport applications.
Challenges
- Energy Intensity: Compressing hydrogen to such high pressures requires significant energy, reducing overall efficiency.
- Safety Concerns: High-pressure systems demand rigorous safety standards to prevent leaks or failures.
- Infrastructure Needs: Widespread adoption requires substantial investment in refueling and storage infrastructure.
Innovations in Compressed Hydrogen Storage
- Advanced Materials: New composite materials, such as carbon fiber reinforced polymers, are reducing the weight and cost of high-pressure tanks while improving safety.
- Smart Sensors: Integrated monitoring systems can detect leaks, pressure changes, or temperature fluctuations in real-time, enhancing safety.
- Cryo-Compressed Systems: Combining high pressure with low temperatures can increase storage density, making this hybrid approach a promising evolution.
💡 Real-World Example: Toyota’s Mirai, a hydrogen fuel cell vehicle, uses compressed hydrogen storage with lightweight carbon fiber tanks, offering a driving range of over 300 miles per refueling.
Key Takeaway:
Compressed hydrogen storage is a foundational technology for the hydrogen economy, but ongoing innovations are critical to addressing its limitations. Advances in materials, safety systems, and hybrid methods are paving the way for safer, more efficient storage solutions.
Liquid Hydrogen Storage – Cooling Hydrogen for Compact Storage
❄️ Liquid hydrogen storage involves cooling hydrogen to extremely low temperatures, creating a dense energy carrier that’s easier to transport and store in large quantities.
How It Works
- Cryogenic Cooling: Hydrogen is cooled to -253°C (-423°F), the point at which it transitions from gas to liquid.
- Storage Tanks: Specialized cryogenic tanks with vacuum insulation are used to maintain these ultra-cold temperatures, minimizing evaporation losses.
Current Applications
- Space Exploration: NASA has long used liquid hydrogen as rocket fuel, showcasing its energy density and reliability.
- Energy Transport: Liquid hydrogen is being tested as a practical solution for exporting renewable energy from regions rich in wind or solar power. For instance, Australia is developing hydrogen export projects using liquid hydrogen to transport energy to Japan.
Advantages
- High Energy Density: Liquid hydrogen is much denser than its compressed counterpart, enabling more storage in the same volume.
- Transport Efficiency: Ideal for long-distance shipping, particularly in large quantities.
- Versatility: Suitable for a variety of applications, from industrial use to power generation.
Challenges
- High Energy Requirement: Liquefaction consumes a significant amount of energy, reducing the overall efficiency of the hydrogen cycle.
- Boil-Off Losses: Even with advanced insulation, some hydrogen inevitably evaporates during storage and transport.
- Infrastructure Costs: Requires specialized facilities and equipment for liquefaction, storage, and distribution.
Innovations in Liquid Hydrogen Storage
- Improved Insulation Technologies: Advances in cryogenic insulation, such as multi-layered reflective materials, are reducing boil-off losses.
- Next-Generation Liquefaction Processes: Research into magnetic refrigeration and other techniques aims to lower the energy cost of liquefaction.
- Hybrid Transport Systems: Combining liquid hydrogen storage with advanced cooling containers for seamless integration into global supply chains.
đź’ˇ Real-World Example:
The Suiso Frontier, the world’s first liquefied hydrogen carrier ship, was launched by Kawasaki Heavy Industries in 2021. It’s part of a pilot project to transport liquid hydrogen from Australia to Japan, demonstrating the viability of large-scale liquid hydrogen storage and transport.
Key Takeaway:
Liquid hydrogen storage offers a compact and efficient way to transport energy across vast distances, making it essential for global hydrogen trade. However, its high energy requirements and infrastructure costs call for continued innovation.
Solid-State Hydrogen Storage – The Future of Compact and Safe Storage
🔬 Solid-state hydrogen storage involves absorbing hydrogen into solid materials, offering an innovative solution for safe and efficient storage at low pressures and moderate temperatures.
How It Works
- Hydrogen Absorption: Hydrogen gas is chemically or physically absorbed into solid materials, such as metal hydrides, porous materials, or chemical compounds.
- Release Mechanism: When heated, these materials release hydrogen gas for use in energy applications.
Current Applications
- Portable Fuel Cells: Solid-state storage is used in small-scale applications like backup power systems or portable electronics.
- Experimental Vehicles: Research initiatives are exploring the use of solid-state hydrogen storage in passenger cars, trucks, and drones.
Advantages
- Safety: Low-pressure storage reduces the risks associated with high-pressure or cryogenic hydrogen systems.
- Compact Storage: Solid materials can hold significant amounts of hydrogen in a small volume.
- Reusability: Many storage materials can absorb and release hydrogen repeatedly without significant degradation.
Challenges
- Material Costs: Advanced materials like metal hydrides or carbon nanotubes can be expensive to produce at scale.
- Energy for Release: Extracting hydrogen from solid-state storage often requires heat, which can reduce system efficiency.
- Slow Kinetics: The speed of hydrogen absorption and release is still a limitation in many materials.
Innovations in Solid-State Hydrogen Storage
- Metal-Organic Frameworks (MOFs): These porous materials have shown tremendous potential for high-density hydrogen storage at room temperature.
- Advanced Hydrides: Alloys like magnesium hydride are being optimized for faster absorption and release cycles.
- Thermal Management Systems: New designs integrate efficient heating systems to reduce energy loss during hydrogen release.
đź’ˇ Real-World Example:
Hydrogenious LOHC Technologies, a German company, has developed a Liquid Organic Hydrogen Carrier (LOHC) system where hydrogen is stored in a chemical compound. While not strictly a solid-state system, it highlights similar principles by making hydrogen storage safer and more compact, demonstrating a practical application of advanced storage materials.
Key Takeaway:
Solid-state hydrogen storage represents a promising path toward safer, more compact, and efficient hydrogen systems. As material science advances, these technologies could become a cornerstone of the hydrogen economy, particularly for portable and decentralized applications.
Underground Hydrogen Storage – Large-Scale Solutions for Energy Resilience
🌍 Underground hydrogen storage involves injecting hydrogen into naturally occurring or man-made geological formations, offering a scalable solution for balancing renewable energy supply and demand.
How It Works
- Geological Formations: Hydrogen is stored in salt caverns, depleted oil and gas reservoirs, or aquifers. These formations provide a stable and secure environment for large quantities of hydrogen.
- Injection and Withdrawal: Hydrogen is injected under pressure and can be retrieved when needed to meet energy demands or provide grid stability.
Current Applications
- Renewable Energy Storage: Underground hydrogen storage is being used to store surplus renewable energy, such as excess wind or solar power, for later use.
- Seasonal Energy Balancing: This method helps manage seasonal fluctuations in energy demand, particularly in colder climates.
Advantages
- Massive Capacity: Geological formations can store vast amounts of hydrogen, making this method ideal for large-scale applications.
- Grid Stability: Stored hydrogen can be converted back into electricity to stabilize grids during periods of high demand or low renewable output.
- Cost-Effective: Once infrastructure is established, storing hydrogen underground is relatively economical compared to other large-scale storage solutions.
Challenges
- Site Suitability: Not all regions have the necessary geological conditions for underground hydrogen storage.
- Leakage Risk: Hydrogen’s small molecular size increases the potential for leaks, requiring careful site selection and monitoring.
- Initial Costs: Developing the necessary infrastructure, including pipelines and injection systems, can be expensive.
Innovations in Underground Hydrogen Storage
- Salt Cavern Development: Advances in cavern construction are improving the capacity and safety of salt-based storage systems.
- Hydrogen Monitoring Technology: Real-time monitoring systems are being developed to detect leaks and ensure operational safety.
- Hybrid Systems: Combining underground hydrogen storage with renewable energy plants to create integrated, efficient energy hubs.
đź’ˇ Real-World Example:
The HyStock Project in the Netherlands uses salt caverns to store hydrogen produced from renewable energy. This large-scale storage system supports the country’s transition to a hydrogen-based energy economy, showcasing the potential of underground storage.
Key Takeaway:
Underground hydrogen storage is a critical enabler of the hydrogen economy, providing the scale needed to store renewable energy and stabilize power grids. With continued advancements in technology and infrastructure, this approach will play a pivotal role in global energy decarbonization.
Hydrogen storage is the linchpin of the clean energy future, with solutions ranging from compressed and liquid storage to solid-state and underground methods. Each approach has its own strengths, challenges, and innovations, making them suited to different applications and scales.
As the hydrogen economy grows, investment in storage technologies will be key to unlocking its full potential. By combining safety, efficiency, and scalability, hydrogen storage solutions are poised to power the transition to a sustainable energy world. 🌟🌍