5 Innovative Wave Energy Conversion Technologies to Watch in 2025
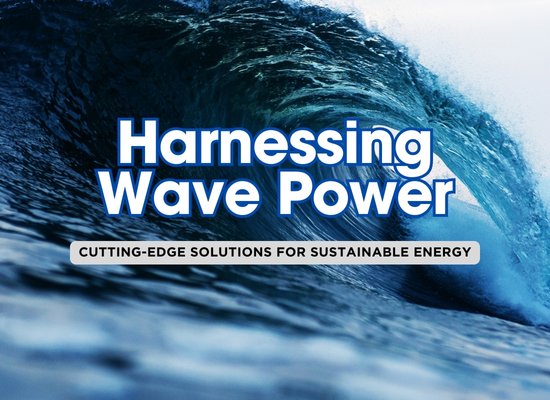
Wave energy is a promising renewable resource, and several innovative technologies are advancing toward commercial viability. Here are five noteworthy wave energy conversion technologies to keep an eye on in 2025:
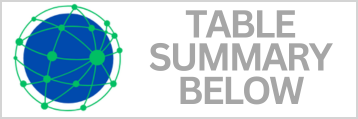
1️⃣ Oscillating Water Column (OWC)
The Oscillating Water Column (OWC) technology harnesses wave energy by using the movement of water to generate air pressure variations. It consists of a partially submerged chamber that allows waves to enter and exit. As the waves rise and fall within the chamber, they push air through a turbine connected to a generator, converting the pressure changes into mechanical energy. This air pressure difference drives a bidirectional turbine, which rotates in both directions to produce electricity. The OWC system can be installed either onshore or offshore, depending on the water depth and wave conditions.
The key aspect of OWC systems is the use of air rather than directly converting water movement into electricity. The compression and expansion of air caused by the movement of water are what drive the turbine, which makes this technology unique compared to other wave energy conversion methods.
Key Features:
- Dual Installation Options:
OWC systems can be implemented either onshore or offshore. Onshore OWCs are built near the shorelines, where waves crash against the coast, causing the air pressure to fluctuate inside the chamber. Offshore OWCs are typically installed on floating platforms, often located in deeper waters where wave patterns are more consistent. This flexibility allows OWCs to be deployed in a range of locations depending on the desired energy output and environmental conditions. - Environmental Integration:
One of the reasons OWCs are considered environmentally friendly is that they do not involve underwater turbines or large-scale construction on the ocean floor. The system operates with minimal disruption to marine life, and the air-driven turbines are generally quieter and less invasive than other underwater systems. - Energy Storage Potential:
Some OWC systems are designed with integrated energy storage capabilities, such as using the generated electricity to pump water into an elevated reservoir. This energy storage method ensures that power can be supplied continuously even when wave conditions are not ideal, making OWCs an excellent option for consistent, renewable energy production.
Recent Developments:
- OE Buoy Technology:
Ocean Energy Ltd. has developed the OE Buoy, a floating OWC device that captures energy from ocean waves. The device consists of a buoyant structure that moves with the waves, generating air pressure variations within the chamber below the water surface. The OE Buoy is considered one of the most promising OWC designs for offshore deployment. Testing of the OE35 buoy, a full-scale version, is set to begin at the U.S. Navy’s Wave Energy Test Site in Hawaii in 2024. - Testing and Trials:
Several other companies and research institutions have been actively testing OWC systems, with some focusing on optimizing turbine efficiency. For example, the Aguçadora OWC project in Portugal is part of a larger effort to demonstrate how onshore OWCs can be scaled for commercial use. These trials have demonstrated that OWC systems can successfully produce electricity in a variety of wave conditions and are continuing to improve in terms of energy conversion efficiency and durability.
Challenges and Considerations:
- Energy Conversion Efficiency:
While OWC systems are effective at harnessing wave energy, optimizing the efficiency of air turbines remains a critical challenge. Researchers are focusing on improving the turbine designs, including switching from conventional turbines to newer, more efficient models that can operate effectively in both directions as the air pressure fluctuates. The overall efficiency of the conversion system must be optimized to make OWC systems commercially viable at large scales. - Maintenance and Durability:
Because OWCs are exposed to harsh marine environments, regular maintenance and ensuring the longevity of the system are crucial challenges. The materials used must withstand saltwater corrosion, high winds, and powerful waves. Offshore OWCs, in particular, require specialized maintenance protocols to ensure minimal downtime.
Why It’s Promising:
OWC systems are considered one of the most scalable and environmentally friendly methods for converting wave energy into electricity. Their ability to be installed both onshore and offshore offers great flexibility, and the use of air turbines makes them a more passive and less disruptive technology. With ongoing research to improve efficiency and reduce maintenance costs, OWC technology has strong potential for contributing to a sustainable, renewable energy future.
2️⃣ Attenuators
Attenuators are long, floating structures designed to capture wave energy by flexing and bending as waves pass. These structures are typically segmented, with each section moving independently. The movement of these sections drives hydraulic pumps or other mechanical systems that convert the mechanical energy into electricity.
Key Features:
- Flexibility in Design: Attenuators are modular, meaning they can be scaled up or down to suit different wave conditions and energy needs. The modular design allows for the creation of larger units that can absorb more energy, making them suitable for various ocean environments.
- Effective in Deep Waters: They are ideal for deployment in deep water, where wave conditions are more consistent and have a greater energy potential. Unlike some other technologies, they don’t require extensive seabed infrastructure.
Recent Developments:
- Testing and Prototypes: Attenuators have been in prototype stages in several countries, including Europe, where they are being tested in real-world conditions to improve energy conversion efficiency. One of the most notable projects includes the Pelamis Wave Power’s prototype, which was tested off the coast of Portugal. These prototypes are designed to capture energy from waves traveling in multiple directions.
Challenges and Considerations:
- Structural Integrity: Because they are long and flexible, attenuators are subject to wear and tear from constant wave action. Engineers continue to refine their designs to improve durability and longevity in harsh marine environments.
- Energy Conversion Efficiency: While the technology has great potential, optimizing the conversion of wave motion into electrical energy remains a significant challenge. Researchers are working on better mechanisms to efficiently capture and convert this energy at scale.
Why It’s Promising:
Attenuators offer a high potential for energy capture from the ocean’s waves, particularly in deep water regions where other technologies may be less effective. Their modular and scalable nature makes them an attractive option for future wave energy systems that could contribute to sustainable energy goals.
3️⃣ Point Absorbers
How They Work:
Point absorbers are buoy-like devices designed to float on the surface of the ocean and capture the energy from the vertical motion of waves. These devices typically consist of a floating buoy that is anchored to the seabed by a column or a rigid structure. As waves pass, the buoy moves up and down, causing relative motion between the buoy and the anchored column. This movement is then converted into mechanical energy through hydraulic pumps, pistons, or direct-drive generators, which convert the mechanical energy into electricity.
The key to point absorber technology is the way it captures energy from the motion of waves. The buoy’s vertical motion, combined with its anchoring system, maximizes energy absorption, allowing for efficient energy conversion even in rougher or deeper waters.
Key Features:
- Compact Design:
Point absorbers are relatively small and can be deployed in various locations, making them an excellent option for areas with space constraints or where other wave energy technologies might not be practical. Their compact nature allows them to be more flexible in terms of installation and scalability. - High Energy Capture Efficiency:
Point absorbers are designed to capture energy from wave motion in all directions, including both vertical and horizontal movement. This makes them particularly effective in areas with multi-directional wave patterns, as they are not limited to just one direction of energy capture. In this sense, they can convert more energy from a given wave condition compared to other technologies. - Adaptability:
Point absorbers are highly adaptable to varying wave conditions. Their design allows them to operate efficiently in both deep and shallow waters, and they can be installed in offshore locations where other types of wave energy devices may not be effective.
Recent Developments:
- AWS Ocean Energy’s “CETO” Technology:
One of the most notable point absorber systems is AWS Ocean Energy’s CETO technology. The CETO device uses an underwater buoy that moves with the waves, driving hydraulic pumps to generate electricity. It has been tested successfully in open-water trials off the coast of Western Australia, where the technology showed promising results. The CETO system can also be connected to an offshore desalination plant, generating not only electricity but fresh water as well, adding extra value to the system. - Full-Scale Testing:
AWS Ocean Energy is currently scaling up its CETO technology with plans for further testing and commercialization. Other companies have also explored point absorbers, including Ocean Power Technologies, which is focused on developing small-scale, commercially viable point absorber systems for both government and private sector customers.
Challenges and Considerations:
- Durability and Maintenance:
Point absorbers, like many wave energy technologies, must contend with the harsh marine environment. Saltwater corrosion, wave action, and biofouling (the accumulation of marine organisms on surfaces) can wear down components over time, leading to higher maintenance costs. As a result, designers are focusing on improving the durability of the materials used and developing easy-to-maintain systems for offshore installations. - Energy Conversion Efficiency:
While point absorbers are effective at capturing energy from wave motion, their efficiency in converting mechanical motion into electricity varies based on the design and wave conditions. Research is ongoing to optimize energy conversion mechanisms, such as pumps, pistons, and turbines, to ensure that point absorbers generate maximum electricity from minimal wave motion. - Deployment and Infrastructure:
Point absorbers must be anchored to the seabed, which can present logistical challenges, particularly in deep or difficult-to-access areas. Additionally, the infrastructure needed for large-scale deployment, including transmission lines and energy storage systems, adds complexity to commercializing this technology.
Why It’s Promising:
Point absorbers are considered one of the most versatile and efficient wave energy conversion systems. Their ability to capture energy from waves in multiple directions and operate in both deep and shallow waters makes them adaptable to a wide range of locations. Furthermore, point absorbers can be used to generate not just electricity but also provide water desalination, which could help address both energy and water scarcity in coastal regions.
4️⃣ Overtopping Devices
How They Work:
Overtopping devices work by capturing incoming waves in a reservoir above sea level. As waves crash over the device, they fill a reservoir that is higher than the sea level, creating stored potential energy. This water is then released through turbines or other mechanisms to generate electricity as it flows back down to the sea. The process is similar to a hydropower dam, where water is stored and then used to generate power when released. Overtopping devices typically consist of a floating platform or a fixed structure that channels the waves into the reservoir.
These devices can store energy during periods of high wave activity, ensuring that power can be generated consistently even when the waves are less active. This makes overtopping devices unique in their ability to provide energy storage in addition to energy generation.
Key Features:
- Energy Storage Capability:
One of the key advantages of overtopping devices is their ability to store energy. Unlike other wave energy technologies, which rely on real-time wave motion, overtopping devices can store energy in the reservoir and release it when needed. This can help provide a steady and reliable power output even when wave conditions are not ideal. - High Efficiency:
Overtopping devices have the potential to achieve high energy conversion efficiency, as the water stored in the reservoir has significant potential energy that can be harnessed. The turbines used to release this water are designed to convert this potential energy into mechanical power, which is then converted into electricity. - Modular and Scalable:
Overtopping devices are scalable, meaning that additional devices can be added to increase energy generation. They are modular in design, allowing for easy expansion or adjustments based on energy needs and available space.
Recent Developments:
- Wave Dragon Project:
One of the most well-known overtopping devices is the Wave Dragon, a project developed in Denmark. The Wave Dragon uses a floating platform with a large “wave collector” that channels water into a reservoir. The system has been tested off the coast of Denmark, and the technology is expected to undergo further trials to refine its design and increase energy generation efficiency. The Wave Dragon has shown promising results, with a pilot project that demonstrated the potential of overtopping devices to harness wave energy at a commercial scale. - Commercial-Scale Testing:
The Wave Dragon team has been working to refine the device’s performance, aiming to demonstrate its feasibility at full scale. The technology is also exploring ways to improve energy capture efficiency and integrate the device with other renewable energy systems for hybrid power generation, such as offshore wind.
Challenges and Considerations:
- Environmental Impact:
While overtopping devices are designed to have minimal environmental impact compared to other wave energy systems, they still pose potential risks to marine life and ecosystems. The large reservoirs and floating structures can affect the local marine environment, and their effects on ocean currents and wave patterns need to be carefully considered and monitored. Additionally, the potential for biofouling (marine organism growth on surfaces) could affect the efficiency and longevity of the devices. - Cost and Infrastructure:
The initial capital investment for overtopping devices is relatively high due to the complexity of building and maintaining large floating platforms and reservoirs. Additionally, there are significant costs associated with the necessary infrastructure, such as underwater cables for transmitting the electricity generated. However, once deployed and optimized, overtopping devices could prove to be cost-effective in the long run, especially as they offer both energy generation and storage capabilities. - Maintenance and Durability:
Like many marine energy technologies, overtopping devices are subject to harsh ocean conditions, including saltwater corrosion, high waves, and extreme weather. Ensuring the durability of the structures and developing low-maintenance designs for offshore installation are key challenges in commercializing this technology. Advances in materials and construction methods are helping to address these issues.
Why It’s Promising:
Overtopping devices offer a unique combination of wave energy capture and energy storage, giving them an advantage over other technologies that rely solely on real-time wave motion. The ability to store energy during high wave periods and release it when demand is higher or wave conditions are less favorable can help provide a more consistent and reliable power supply. With further testing and development, overtopping devices could become a key player in the transition to renewable energy.
5️⃣ Submerged Pressure Differential Devices
How They Work:
Submerged Pressure Differential Devices (SPDD) capture the energy of waves by utilizing the natural pressure differences between the crest and trough of waves. These devices are typically located below the water’s surface, where the pressure changes are more pronounced. As waves pass over the device, the pressure difference between the high and low points of the waves is harnessed to drive turbines or generate mechanical motion. The pressure differential is then converted into electricity using various mechanisms, such as hydraulic pumps or direct-drive generators.
What makes SPDDs unique is that they operate submerged, making them less visually disruptive and potentially more durable than surface-mounted systems. These devices capture energy from the pressure changes rather than the motion of the water, which can allow for more stable and consistent energy generation.
Key Features:
- Submerged Design:
One of the main advantages of SPDDs is their submerged nature. Because they are placed below the water’s surface, they have a lower visual and physical impact on the environment compared to surface devices. This can make them more appealing for areas with strict environmental or aesthetic regulations. Additionally, being submerged helps protect the device from harsh weather conditions and reduces wear and tear from high winds and rough sea states. - Efficient Energy Capture:
By capturing the pressure difference between the crest and trough of waves, SPDDs can convert energy with high efficiency, particularly in areas where wave height variations are significant. This method of capturing energy can also be more consistent, as it doesn’t rely on the specific movement of water but on the constant pressure changes that occur with each wave. - Adaptability to Different Depths:
SPDDs are versatile in terms of where they can be deployed. Since they are designed to be submerged, they can operate effectively in both deep and shallow waters. Their deployment doesn’t require significant seabed installation, making them easier to install compared to other wave energy technologies that require anchoring to the seafloor.
Recent Developments:
- Prototype Testing:
SPDDs are still in the research and development phase, but prototype devices have been tested in various locations to assess their performance. These prototypes are generally small-scale devices designed to prove the concept before scaling up to commercial-sized units. The ongoing tests are focused on optimizing energy capture efficiency, improving the durability of the devices, and evaluating their long-term performance in real-world ocean conditions. - Collaborations with Offshore Energy Projects:
Several offshore energy projects are looking into integrating SPDDs into their energy systems. They are being considered not only for wave energy capture but also for their ability to integrate with existing offshore wind farms, potentially creating hybrid systems that maximize renewable energy output. These hybrid systems could help stabilize energy generation by combining wind and wave power, which can be complementary sources of energy.
Challenges and Considerations:
- Environmental Impact:
While submerged devices are less visually invasive, they still pose some environmental risks. The noise generated by pressure differentials could potentially affect marine life, and there is the possibility of disruption to ocean currents and local ecosystems. Careful environmental assessments are necessary before large-scale deployment. - Durability and Maintenance:
As with any underwater device, SPDDs face challenges related to durability. The pressure, saltwater, and biological fouling (organisms growing on the devices) can degrade materials over time. Developing long-lasting materials and easy maintenance systems will be crucial to making SPDDs viable for large-scale commercial use. - Cost of Development:
The initial development cost of SPDDs, including research, testing, and infrastructure, can be high. However, the cost may decrease over time as the technology matures and mass production techniques are developed. In the long run, if SPDDs prove to be an efficient and reliable energy source, they could offer competitive costs compared to other forms of renewable energy.
Why It’s Promising:
Submerged Pressure Differential Devices present a promising solution for harnessing wave energy in a way that is both efficient and minimally disruptive to the environment. By using pressure differences rather than water movement, they provide a consistent energy capture method that can work effectively in varying sea conditions. As research and testing continue to improve efficiency and durability, SPDDs could become a key player in the renewable energy sector.
Wave energy continues to show tremendous promise as a sustainable power source, with various technologies evolving to meet the world’s energy demands. Each of the five technologies discussed—Oscillating Water Columns, Attenuators, Point Absorbers, Overtopping Devices, and Submerged Pressure Differential Devices—offers unique advantages in terms of efficiency, scalability, and environmental impact. As advancements are made in these technologies, they will likely play an increasingly important role in the global transition to renewable energy, helping to power homes and industries with clean, sustainable energy drawn from the world’s oceans.
Article Summary
WorldEnergyReport: Wave Energy Conversion Technologies | ||||
---|---|---|---|---|
Technology | How It Works | Key Features | Recent Developments | Challenges |
Oscillating Water Column (OWC) | OWC uses water movement to create air pressure variations inside a chamber, driving a turbine to generate electricity. | Dual installation (onshore or offshore), minimal environmental impact, adaptable to varying wave conditions. | Full-scale testing (OE Buoy) at U.S. Navy Wave Energy Test Site, ongoing research to optimize turbine efficiency. | Efficiency of air turbines, durability in harsh environments, high maintenance costs, environmental integration. |
Attenuators | Floating multi-segmented structures move with the waves, generating energy through hydraulic pumps or mechanical systems. | Modular and scalable, effective in deep waters, captures energy from multi-directional waves. | Prototype testing in Europe (Pelamis and CETO), hybrid systems with offshore wind, scaling up for commercial use. | Durability, wear from constant wave action, optimizing energy conversion efficiency. |
Point Absorbers | Buoy-like devices float on waves, capturing energy through vertical motion, converting it via hydraulic systems or generators. | Compact design, adaptable to various wave conditions, operates in both shallow and deep waters. | AWS Ocean Energy’s CETO technology tested successfully in Australia, plans for further commercial deployment. | Energy conversion efficiency, maintenance challenges in remote marine locations, scaling for commercial use. |
Overtopping Devices | Captures waves in a reservoir above sea level; released water powers turbines to generate electricity. | Energy storage capability, high efficiency, modular design, suitable for variable wave conditions. | Wave Dragon project in Denmark, testing and optimization, energy capture from stored water to ensure steady supply. | Environmental impact, high initial costs, potential disruption to marine life, maintenance of large structures. |
Submerged Pressure Differential Devices | Uses the pressure difference between the wave crest and trough to drive turbines or other mechanical systems. | Submerged design (minimal visual impact), high energy capture efficiency, operates in deep and shallow waters. | Prototype testing in various locations, integration with hybrid offshore wind systems, focus on improving performance. | Environmental impact of underwater noise, durability and wear in harsh conditions, high development costs. |